Can Flame Retardant Catch Fire?
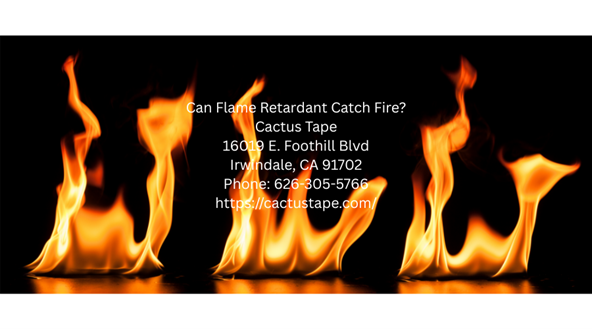
Flame retardants are designed to inhibit combustion and delay fire spread in materials. However, under extreme conditions or when exposed to high-intensity flames, even flame-retardant-treated materials can eventually catch fire. The effectiveness diminishes with factors like the size of the ignition source and material degradation over time. While they work by cooling, forming barriers, or neutralizing radicals, no material is fireproof. For a deeper insight into their performance and limitations, much remains to be explored.
Flame Retardants and Fire Behavior
Although flame retardants are widely utilized in reducing fire hazards, their mechanisms of action are varied and complex. Flame retardant technology leverages several strategies to conform to rigorous fire safety standards. One approach involves cooling effects; materials like aluminum hydroxide release water vapor upon heating, which absorbs heat and lowers material temperature. Additionally, some additives promote char formation, creating carbonaceous layers that block ignition sources and insulate underlying substrates. Endothermic reactions, as seen with ammonium polyphosphate, absorb energy, decreasing the fuel’s temperature. Physical barriers formed by residues like alumina further insulate burning surfaces. In the gas phase, halogenated agents interfere with combustion by neutralizing reactive radicals. These diverse mechanisms collectively enhance the material’s resistance to fire, aligning with established safety protocols.
Effectiveness and Limitations
While flame retardants offer notable benefits in fire prevention, their effectiveness varies considerably across applications. Regulatory standards like TB 117 guarantee that flame-retarded products exhibit reduced fire spread, delaying ignition and slowing fire progression. However, real-world impact appears negligible, with minimal differences in ignition times for consumer products. Despite reducing heat release and toxic gas production, these chemicals often increase smoke toxicity compared to natural materials. Health risks associated with compounds like PBDEs and TDCPP, including cancer and endocrine disruption, raise significant concerns. Moreover, the environmental persistence of these substances leads to global contamination, impacting ecosystems. Combustion processes release toxic byproducts, posing long-term health risks for firefighters and occupants, highlighting the need for safer material innovations.
Mechanisms of Action
Examining the mechanisms of action for flame retardants reveals the intricate processes that underlie their fire-suppressing capabilities. Flame retardants leverage chemical interactions to enhance thermal stability and reduce material flammability. Endothermic degradation plays a significant role, where hydrates like aluminum and magnesium hydroxides release water upon heating. This process absorbs heat, redirecting the exothermic energy of combustion to endothermic reactions, effectively lowering substrate temperatures below ignition points. The high thermal stability of these compounds guarantees they degrade at ideal temperatures, protecting the underlying polymers. By transferring thermal energy to the water release, these interactions inhibit ignition and contribute to the material’s overall fire resistance, showcasing the complexity and efficacy of flame retardant systems in preventing fire propagation.
Real-World Performance Factors
Understanding the real-world performance of flame retardants requires an analysis of factors that influence their effectiveness in preventing fire ignition and spread. In real-world scenarios, flame-retarded materials can prevent ignition from small open flames but may not withstand larger ignition sources. Polyurethane foam with retardants burns 25% slower than its non-retarded counterpart, yet high-intensity flames can still breach barriers. Endothermic retardants like magnesium hydroxide absorb heat, reducing combustion intensity, but may not suffice under extreme conditions. Fire spread correlates with ignition source size, and char formation decreases combustion efficiency. Toxic gas release remains a concern; for instance, polyurethane foams emit high CO levels regardless of retardants. Consequently, integrating systemic fire protection is essential for effective fire risk management.
Material-Specific Behavior
Some materials exhibit unique behaviors when treated with flame retardants, affecting their ability to catch fire. Different flame retardant types, such as those used for textiles or polymers, interact distinctively with the substrate. Inherently flame-retardant materials, like wool and certain synthetic fibers, resist ignition and form protective char layers, contributing to their effectiveness under moderate ignition conditions. However, they may still combust under extreme heat.
Chemically treated fabrics release non-combustible gases to inhibit ignition, but these treatments can degrade over time, reducing their fire resistance. Magnesium and aluminum hydroxides, utilized for their endothermic degradation properties, can fail under excessive heat, leading to potential combustion. Ultimately, the material’s inherent properties and the applied flame retardant type dictate its behavior under fire exposure.
Key Research Findings and Controversies
Although flame retardants play an essential role in fire mitigation, their effectiveness and associated risks ignite ongoing debates in scientific circles. Flammability tests reveal that while these chemicals slow combustion by disrupting the fire triangle, they don’t prevent ignition entirely. Critics highlight that flame retardants often delay fire ignition by mere seconds, raising questions about their genuine fire safety benefits. Despite regulatory challenges, such as balancing fire safety with health concerns, studies indicate that flame-retarded materials can reduce smoke mass and combustion products. Nonetheless, minimal differences in smoke toxicity are noted between treated and untreated materials. Additionally, toxicological concerns persist, particularly with halogenated and organophosphorus compounds linked to significant health risks, contributing to broader controversies surrounding their use.
Applications in Different Industries
Flame retardants are integral across various industries, each demanding specific fire safety standards and applications. In electronics, brominated flame retardants are essential in printed circuit boards (PCBs) for devices like TVs and computers, ensuring compliance with stringent industry regulations. The automotive sector benefits from flame retardant innovations in electric vehicle components, such as battery pack casings and wiring systems, to meet safety standards. Building and construction industries incorporate flame retardants in concrete, insulation, and panels to adhere to fire safety codes. Textiles employ these compounds in fire-resistant fabrics for high-risk environments, including military and firefighting gear. Other applications include aerospace and coatings, where halogen-free alternatives address eco-conscious industry demands, reflecting a significant market trend toward sustainable flame retardant solutions.
Environmental and Health Considerations
While flame retardants play an essential role in enhancing fire safety across various industries, their environmental and health implications can’t be overlooked. Flame retardants persist in environments, leading to significant bioaccumulation risks. They’re detected in human biomarkers, including breast milk and blood, indicating absorption into tissues. This persistence also facilitates global transport, impacting even remote ecosystems like the Arctic. Health-wise, certain brominated compounds disrupt endocrine function, causing thyroid dysfunction and hormone interference. Long-term exposure has been linked to neurodevelopmental issues, reproductive toxicity, and potential carcinogenicity. Additionally, flame retardants’ aquatic toxicity threatens ecosystems, with harmful effects on fish and amphibians. Regulatory challenges persist, as replacements often mirror the toxicity of phased-out substances, complicating efforts for safer alternatives.
Frequently Asked Questions
Are Flame Retardants Environmentally Friendly?
Flame retardants aren’t environmentally friendly due to sustainability concerns and significant ecological impact. They persist in ecosystems, bioaccumulate in food chains, and pose health risks. Regulatory efforts aim to mitigate these issues by promoting safer, non-toxic alternatives.
What Are the Most Common Types of Flame Retardants?
The most common flame retardants include halogenated compounds like TBBPA and PBDEs, and non-halogenated options such as organophosphates. Halogenated compounds act by radical quenching, while non-halogenated options form a protective char layer, insulating materials effectively.
How Do Flame Retardants Impact Indoor Air Quality?
Flame retardants degrade indoor air quality by releasing toxic pollutants. They leach from household items like furniture and electronics, increasing toxicity in indoor environments. These pollutants pose health risks, especially in confined spaces with high concentrations.
Can Flame Retardants Be Safely Used in Children’s Products?
Flame retardants pose significant risks in children’s products despite safety regulations. Chemical exposure from these substances can lead to serious health issues. Evaluating alternatives and enforcing stricter regulations is essential for ensuring children’s safety and reducing potential harm.
Are There Alternatives to Traditional Flame Retardants?
The current question asks if there are alternatives to traditional flame retardants. Yes, there are plant-based options and natural fibers, utilizing renewable resources. They’re designed to lower toxicity and environmental impact, enhancing sustainability in various applications.